
Design of Spillway Shape that Contributes to Dam Rehabilitation
In Japan, with development of dams from both perspectives of flood control and water utilization, dams play a major role as one form of social capitals supporting social and economic activities. In the future, dams are expected to play an increasingly important role due to the effects of climate change, such as more intensive rainfall.
Under these circumstances, additional spillways and dam heightenning are implemented for various reasons, such as changes to flood control and water utilization plans.
In particular, when improving or enhancing spillways with relatively large discharge capacity, the following methods are typically used: (1) improvement or extension of the spillway at the top of the dam, (2) improvement or extension of the spillway of the dam body, and (3) construction of a new tunnel spillway(Figure 1).
The Hydraulics and Sediment Transport Engineering Team designs the shape of dam spillways (hydraulic design) in case of (1) to (3). Some examples are introduced below.
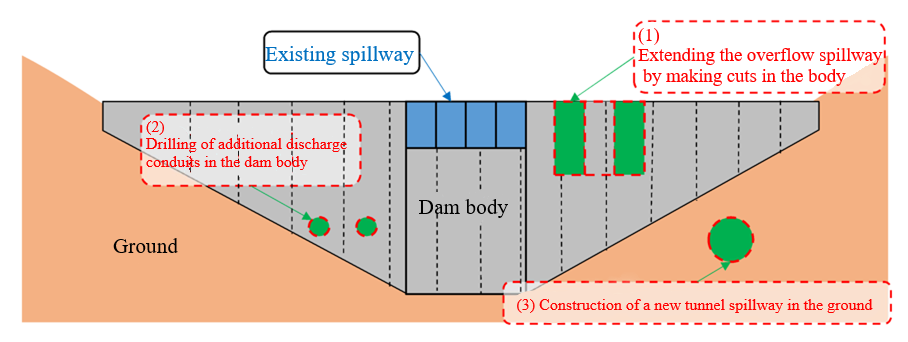
(1) Improvement or extension of the spillway at the top of the dam
A typical example of a large-scale improvement or extension of the spillway at the top of the dam is the modification of the Nagayasuguchi Dam.With this modification, a portion of the top of the dam on the right bank side was cut into U-shape(a depth of approximately 37 m; the deepest ever so far), to install two spillway gates, with the aim of enhancing the flood control function.
Since up to 4,000 m3/s of extra water would be merged into the existing spillway flow from the right bank side of the energy dissipator, hydraulic model experiments were conducted before designing the shape of the structure to confirm whether a stable flow is formed with safe floodwater discharge (Figure 2).
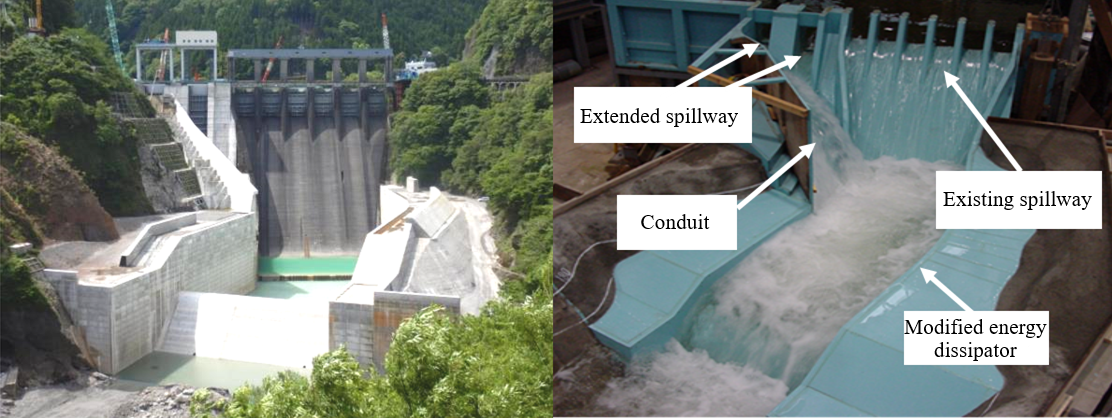
Figure 2 Nagayasuguchi Dam after modification and hydraulic model experiment
(Photos courtesy of the Nakagawa River Office, Shikoku Regional Development Bureau)
(2) Improvement or extension of the spillway of the dam body
A typical example of a large-scale dam-body spillway improvement or extension is the modification of the Tsuruda Dam. With this modification, three wide 5 m × 5 m holes were drilled in the dam to install additional discharge conduits, thus enhancing the flood control function.
Since the discharge conduits have curved sections, the conduits might be damaged due to local pressure descent. Therefore, pressure inside the conduits was measured for safely designed shape.
In addition, the discharge from additional conduits merges into the existing spillway flow from the right bank at the downstream river channel (Figure 3 ).Therefore, hydraulic model experiments were conducted before designing the shape of the structure to confirm the stablity and safety of discharge flow conditions.
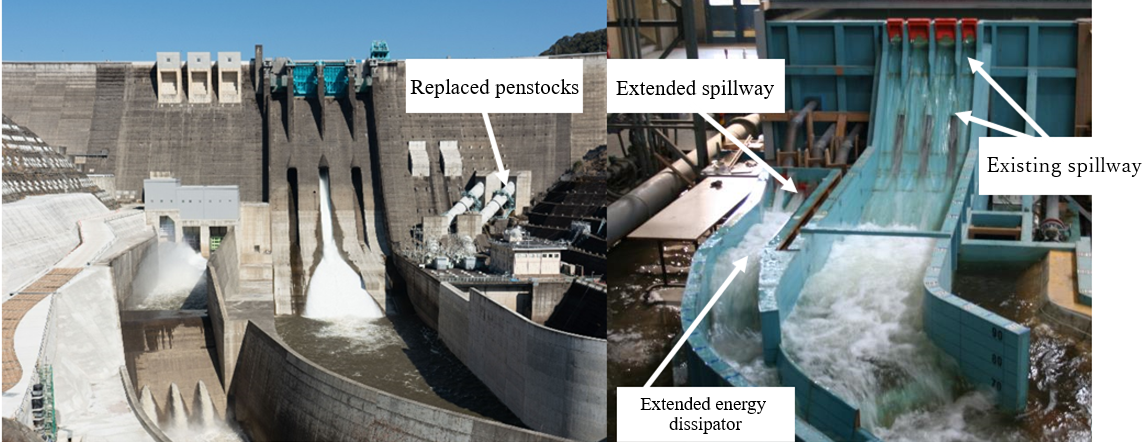
Figure 3 Tsuruda Dam after modification and hydraulic model experiment
(Photos courtesy of the Sendaigawa River Office, Kyushu Regional Development Bureau)
(3) Construction of a new tunnel spillway
A typical example of constructing a new large-scale tunnel spillway is the modification of the Kanogawa Dam. This modification involves the construction of a new tunnel with a diameter of 11.5 m and a length of 457 m to allow approximately 1,000 m3/s of additional discharge from the tunnel.
The additional discharge was merged into the existing spillway flow from the right bank. In this case, there were site constraints between the extended energy dissipator and the river channel. Therefore, steps and bars (baffle piers) were installed in the energy dissipator to create a compact structural shape(Figure 4) .
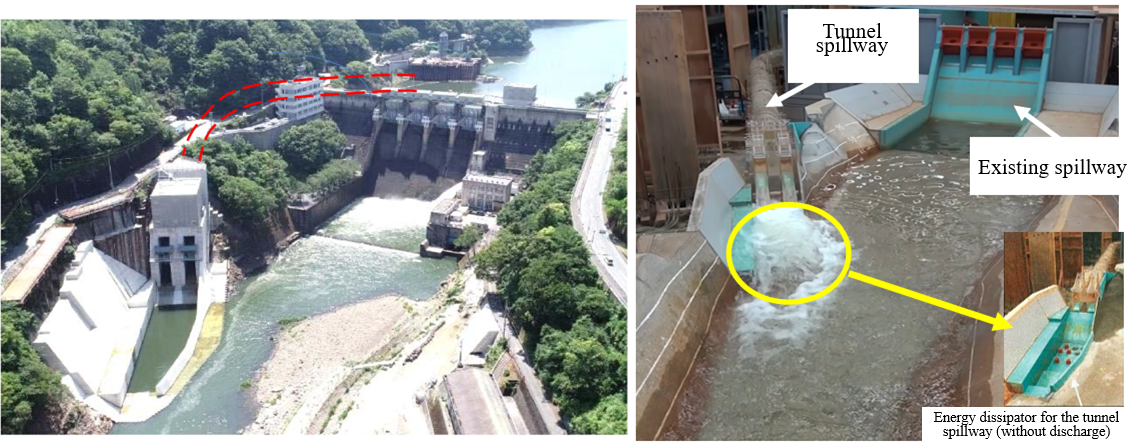
Figure 4 Kanogawa Dam after modification and hydraulic model experiment
(Photos courtesy of the Yamatosaka Dam Construction Office, Shikoku Regional Development Bureau)
As described above, the Hydraulics and Sediment Transport Engineering Team will continue to apply hydraulic model experiments and other methods to contribute to the design of dams for safe management of floods in response to an expected increase in the number of dam modifications (dam rehabilitation projects) in future.
(Contact : Hydraulics and Sediment Transport Engineering Team)
Salt Content Survey of Concrete Bridges Utilizing Non-Destructive Testing Equipment
Salt damage is one of the most serious deterioration factors involved in concrete bridges, and occurs very frequently. Accordingly, it is necessary to promote preventive maintenance by detecting the signs of deterioration and taking appropriate measures.
When conducting salt damage surveys on concrete bridges, concrete cores are typically collected on-site and measured for salinity indoors.
However, the process involves such issues as damage to the structure due to core sampling, limited measurement points, and time-consuming salinity measurement.
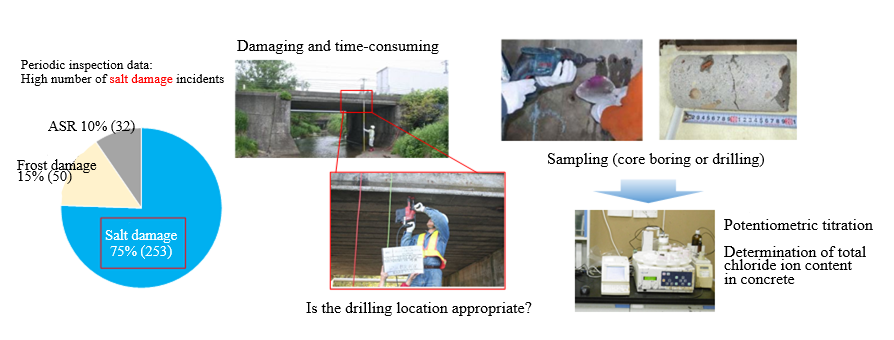
Figure 1 Conventional salt survey of concrete bridges
The detected salinity is not necessarily representative of the bridge, since the amount of salt varies greatly depending on the location where the concrete core was taken, as well as the environmental conditions and structural type. Thus, it is necessary to develop a screening method to find representative areas of salinity from the entire structure at an early stage, which can then be used for preventive maintenance measures.
This study therefore utilizes actual bridges that have deteriorated due to salt damage to propose a survey method using non-destructive testing equipment that can measure salinity on-site and enable efficient surveys.
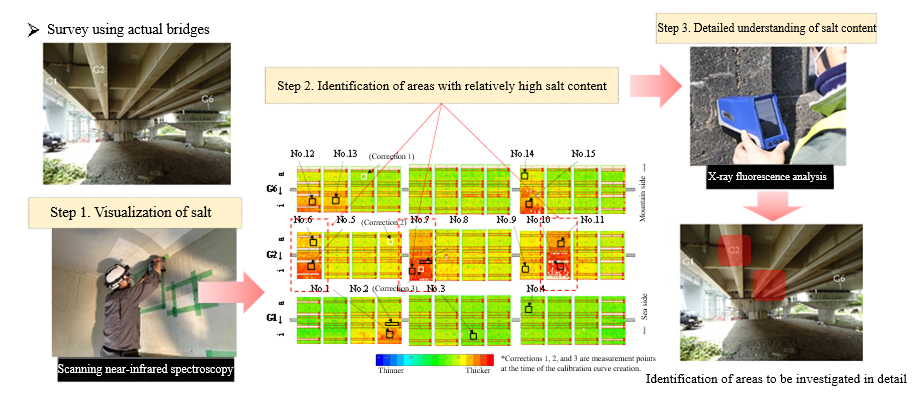
Figure 2 Visual representation of an efficient method for surveying the salt content using a non-destructive testing technique
With the survey, the amount of salt adhering to the entire structure is roughly determined by scanning near-infrared spectroscopy, which uses the principle of near-infrared light (irradiating light and spectroscopy of the reflected light to determine the amount of salt elements based on the absorbance at each wavelength). Then, by applying the X-ray fluorescence analysis method, based on the principle of X-ray fluorescence (identifying the amount of salt elements based on the energy of the X-ray fluorescence generated by X-ray irradiation) for areas with high salt content,
it is possible to screen the areas with a maximum total chloride ion content within the measurement range on-site and to reasonably select areas where preventive maintenance measures should be implemented.
We plan to study even more efficient non-destructive testing techniques and verify non-destructive testing techniques using actual bridges to resolve issues with on-site implementation, such as the detection range, accuracy, and ease of handling in the future.
(Contact : Bridge and Structural Engineering Research Group)
Study of Maintenance Techniques for River Structures with Accelerated Combined Deterioration
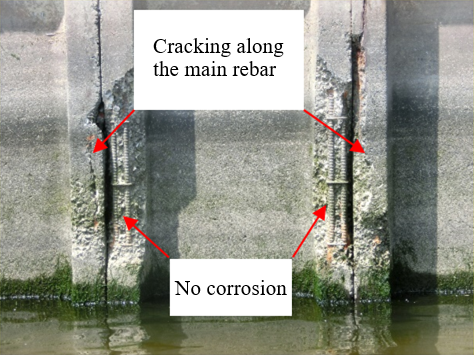
Fig. 1 Deterioration of the convex parts
of on-site concrete sheet piles
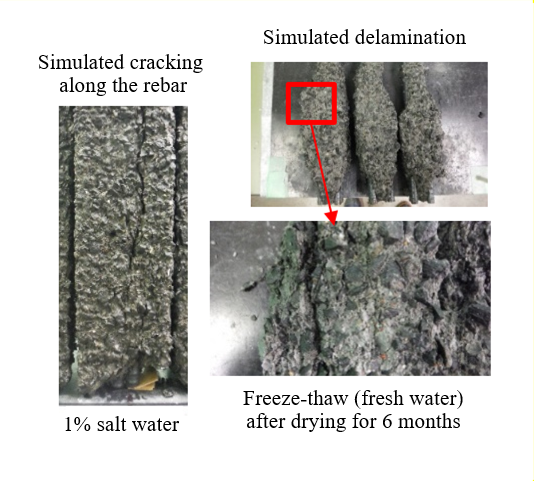
Fig. 2 Simulation test conditions
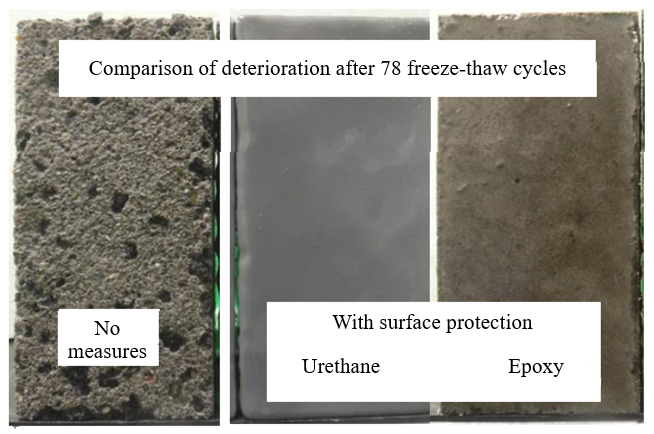
Fig. 3 Surface protection as a measure
to improve resistance to frost damage
1.Combined deterioration of river structures
Concrete structures in cold region rivers are subject to combined deterioration due to frost damage and collision/abrasion with river ice and other substances.A concrete sheet pile revetment constructed 20 years earlier near a river mouth showed concrete delamination, exposed rebar and other apparent deterioration.(Fig.1)
2.Survey of concrete sheet pile revetment deterioration
The above-mentioned deterioration of concrete sheet piles had progressed on the convex parts of the sheet piles to almost the same height of the water surface at low tide, but the exposed rebar did not rust, indicating that it was unlikely that concrete delamination was due to salt damage deterioration as a result of rebar corrosion. River ice colliding with the concrete sheet piles was observed in a winter survey, and concrete delamination often extended deeper than where the rebar was located. It was accordingly concluded that a factor behind this was deterioration preceded by frost damage.
A concrete sheet pile section, which had been repaired shortly before with a heavy-duty formwork method using ultra-high strength fiber reinforced concrete was in a good condition without deterioration or damage for four years after the repair work; however, the high repair cost is an issue to be solved. In another section that had previously been repaired using a carbon-fiber sheet method, numerous spalls from protective mortar joints were observed three years after the repair work.
3.Laboratory deterioration simulation tests
Freeze-thaw tests were performed using specimens made of concrete with low air content and restrained by rebar, as in case of the concrete sheet piles on site, to verify the deterioration causes. Based on the tests, cracks in the direction parallel to the main rebar rods were created by expansion in the volume of the concrete, and the amount of delaminated concrete increased in a test using 1% salt water, which was close to the measured salinity of the on-site water when it was compared with a test using fresh water. An additional freeze-thaw test was also conducted on specimens that had been produced by normal curing under water and then drying for six months, because deterioration was more prevalent in the sections of the concrete sheet piles that underwent repeated on-site drying and wet conditions than in sections that were continuously under on-site wet conditions. It was confirmed that the amount of delamination was greater and the deterioration appeared to be more similar to the actual on-site deterioration in the latter than the former test.(Fig.2)
4.Study of deterioration control measures
A one-sided freeze-thaw test was performed in 1% salt water on specimens treated with two types of surface protection materials to verify their effectiveness and durability because intercepting water is effective in preventing concrete sheet pile deterioration preceded by frost damage. As a result, it was confirmed that protection of the surface with polyurethane resin or epoxy resin significantly increased frost damage resistance and turned out to be a very effective measure to prevent deterioration preceded by frost damage.(Fig.3)
5.Conclusion
We will continue our study so that site-specific measures can be proposed to control various combinations of combined deterioration of concrete structures.
(Contact : Materials Research Team, CERI)